Understanding how our organs produce energy efficiently is crucial, and substrate selection plays a vital role in this process. Each organ has its preferred fuel source: the heart, for instance, relies on fatty acids, while muscles prioritize glucose. Tools like Metabolic Control Analysis help us determine the impact of different enzymes on metabolism. Proper regulation of pathways involving glucose and fatty acids, such as through PDH activity, is critical. Computational models simulate these processes, offering valuable insights. Future research aims to better quantify and control these pathways. By examining these elements, we can gain a deeper understanding of metabolic regulation.
Key Takeaways
Different organs have specific fuel preferences for optimal metabolic efficiency. For instance, cardiomyocytes thrive on fatty acids, while skeletal muscles rely on glucose.
Metabolic Control Analysis (MCA) is a valuable tool for identifying the enzymes that regulate the balance between glucose and fatty acid metabolism.
Pyruvate Dehydrogenase (PDH) plays a critical role in determining whether glucose or fatty acids are oxidized for energy.
Computational models simulate the impact of substrate levels on metabolic pathways, revealing key regulatory control points.
Future research aims to refine these computational models and uncover new regulatory elements affecting substrate selection in metabolism.
Importance in Metabolism
Understanding substrate selection in metabolism is critical because it directly influences how organs produce and utilize energy. Each organ has its own fuel preference, which affects its metabolic efficiency. For example, cardiomyocytes primarily rely on fatty acids, leveraging their high-energy yield to maintain heart function. In contrast, skeletal muscles prioritize glucose, especially during high-intensity activities, ensuring rapid energy availability.
Substrate selection isn't just about preference; it's about the convergence of catabolic pathways at AcCoA production. This convergence is vital for energy metabolism and signaling. Glucose and fatty acids reciprocally control these pathways, with enzymes like phosphofructokinase and pyruvate dehydrogenase playing key roles in regulating substrate utilization. These enzymes decide whether glucose or fatty acids will dominate in energy production.
Computational modeling helps us better understand substrate selection by simulating steady-state behavior and flux variations in pathways like glycolysis and mitochondrial oxidation. These models enable us to dissect the regulatory mechanisms underlying substrate selection. By optimizing substrate selection, we can potentially improve organ function and overall metabolic health, aligning with our goal of greater freedom in health management.
Metabolic Control Analysis
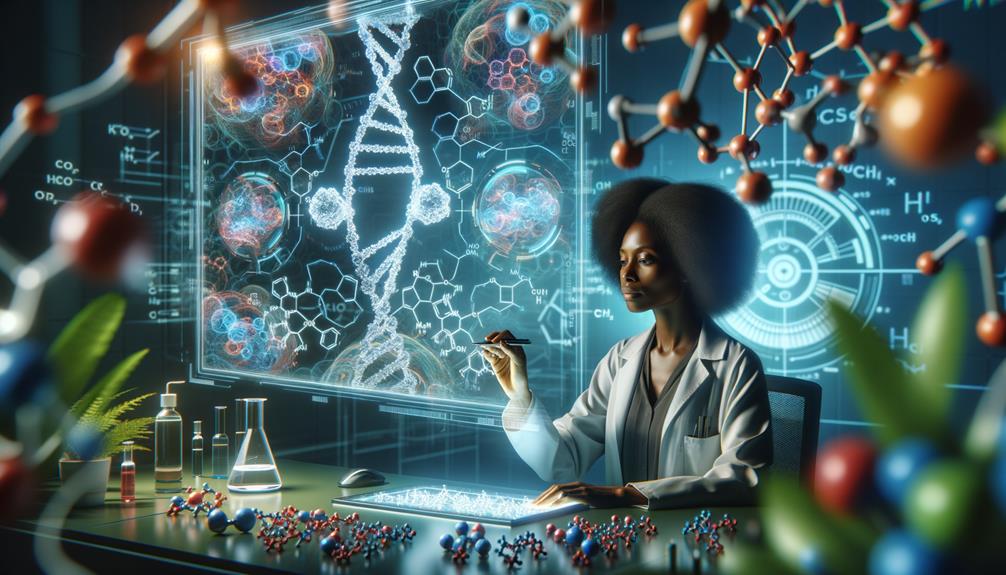
Metabolic Control Analysis (MCA) provides a quantitative understanding of how changes in enzyme activity impact overall metabolic fluxes, offering valuable insights into metabolic regulation. By calculating flux and metabolite concentration control coefficients in metabolic networks, MCA helps us understand how a substrate increase influences the entire metabolic pathway. This approach is crucial for optimizing biotechnological processes and formulating disease prevention strategies.
Highly regulated enzymes, such as phosphofructokinase (PFK) and pyruvate dehydrogenase (PDH), play critical roles in controlling central catabolism. When substrate levels increase, these enzymes adjust their activity, impacting the flux through glycolysis and mitochondrial oxidation pathways. MCA identifies which enzymes exert the most control, thereby identifying potential targets for metabolic engineering.
Computational modeling allows us to simulate the effects of varying substrate levels and enzyme activities on metabolic flux. This helps us understand how substrate selection is regulated and how metabolic pathways adapt to changes in substrate availability. MCA provides a detailed map of metabolic control, giving us a strategic advantage in both research and practical applications. By applying MCA, we can better understand the complexities of metabolic networks and achieve desired outcomes in health and industry.
Catabolic Network Regulation
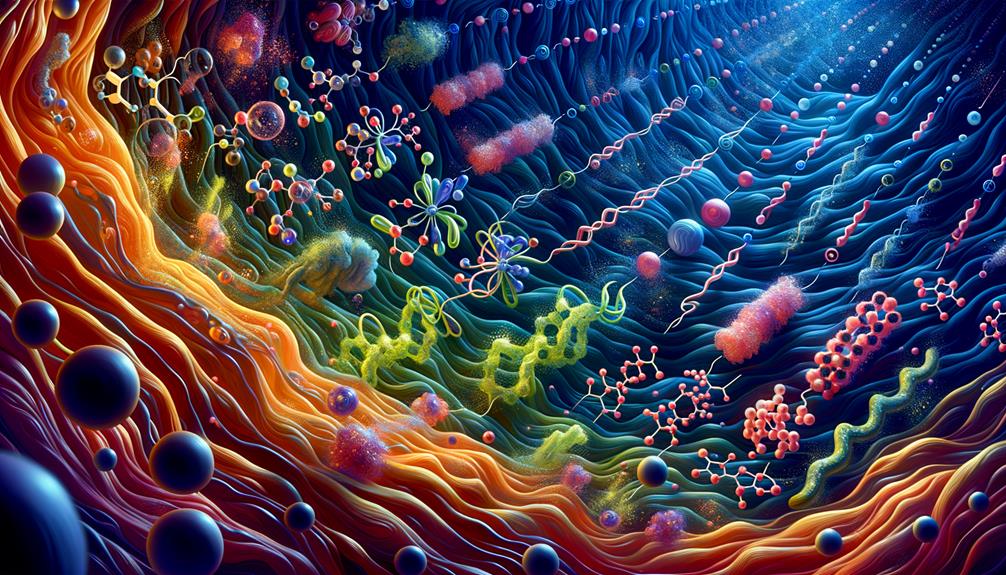
In catabolic network regulation, the delicate balance between glucose and fatty acids determines the metabolic pathways cells prioritize. Enzymes like PDH (pyruvate dehydrogenase) play a crucial role in this process, deciding whether glucose gets converted into acetyl-CoA or if the cell favours fatty acid oxidation.
PDH and other enzymes, such as PFK (phosphofructokinase), exhibit distinct control behaviours in substrate selection. Several key factors influence this process:
Glucose levels significantly impact PDH activity, with high glucose levels increasing PDH activity and promoting glucose oxidation. Conversely, high fatty acid levels inhibit PDH, shifting the cell towards fatty acid metabolism.
Enhanced glucose uptake typically directs the catabolic pathway towards glycolysis and glucose oxidation.
Cells prioritize substrates that meet their immediate ATP demands, which in turn influences PDH activity.
Proper regulation of PDH and other enzymes is vital for efficient energy metabolism and overall metabolic well-being.
Understanding PDH's role in regulating glucose and fatty acid consumption is critical for maintaining metabolic flexibility. This intricate control mechanism allows cells to adapt to various energy demands, promoting a balanced and effective metabolic state.
Computational Modeling
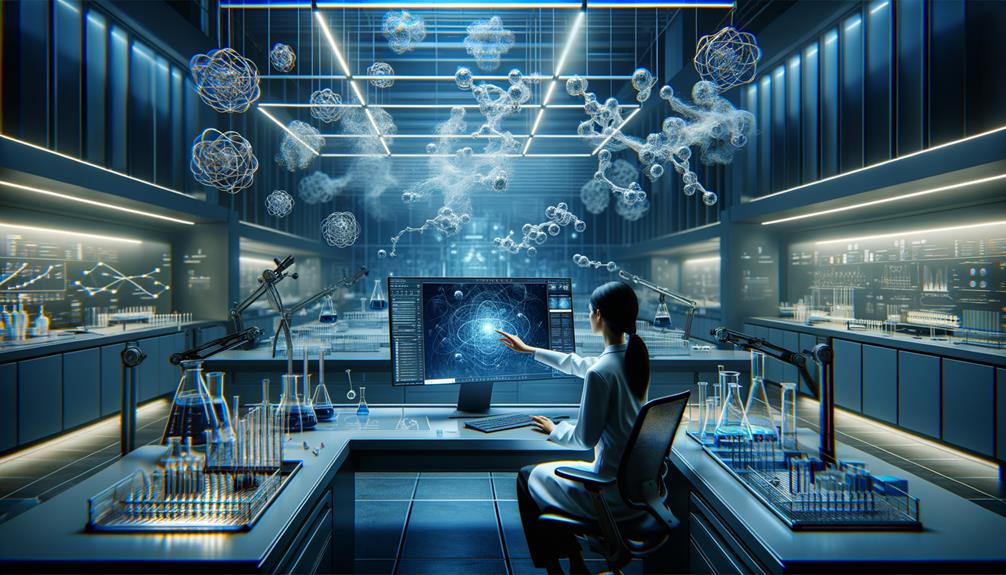
To gain a deeper understanding of how cells balance glucose and fatty acid oxidation, we use computational modeling to simulate central catabolic processes like glycolysis and mitochondrial oxidation pathways. This approach helps us understand how different substrates influence cellular metabolism. By programming detailed rate equations and state variables into Matlab, we analyze the metabolic responses to varying concentrations of glucose and pyruvate CoA (PCoA).
Our simulations focus on the steady-state behavior of these pathways, particularly the flux through pyruvate dehydrogenase (PDH). By adjusting substrate levels, we observe how cells modulate their catabolic processes to maintain energy homeostasis. This model sheds light on the glucose-fatty acid cycle and provides insight into the delicate balance between carbohydrate and lipid metabolism.
Through these computational models, we gain insight into how cells prioritize substrate utilization under different conditions. Precise simulation of glycolysis, glycogenolysis, and mitochondrial oxidation pathways helps us predict metabolic outcomes and identify potential points of regulatory control. This analytical approach provides a robust framework to explore the complexities of substrate selection in cellular metabolism.
Future Research Directions
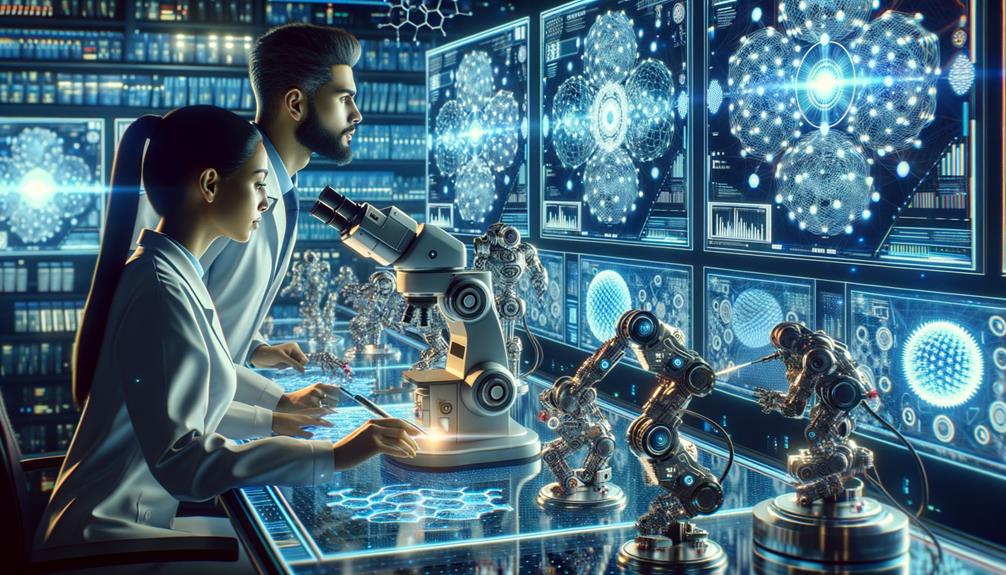
Future research will focus on integrating and quantifying approaches to understand the complexities of catabolic pathways and substrate selection. Building on previous findings, I believe there's a clear path forward in identifying effective strategies for advancing our understanding and application of metabolic control. A primary goal will be to identify specific targets for pharmacological interventions that can effectively modulate substrate selection.
To achieve this, we'll pursue several key areas:
- Metabolic Control Analysis (MCA): By applying MCA, we can gain critical insights into how caloric restriction and time-restricted feeding optimize metabolic pathways.
- Uncharted Regulatory Mechanisms: Investigating new regulatory elements in substrate selection will provide fresh perspectives on central catabolism.
- Diverse Cell Types and Tissues: Applying our findings across various cell types and tissues will broaden our understanding of central catabolism's role in different physiological contexts.
- Integrative Computational Models: We'll refine our computational models to incorporate more complex interactions within catabolic pathways, building on previous modeling efforts.
Frequently Asked Questions
What Is Substrate Selection?
Substrate selection is a crucial step in ensuring the success of scientific experiments or industrial applications. It involves identifying the right compounds that react efficiently with other elements to achieve desired outcomes. By doing so, researchers and manufacturers can optimize their processes, reduce waste, and increase productivity.
What Is a Substrate in Biology?
A substrate in biology is a specific molecule that fits into an enzyme's active site, triggering a chemical reaction. This binding process determines the type of reaction that occurs in biological systems, ensuring enzymes act with precision.
Are Enzymes Substrate Specific True or False?
Enzymes are remarkably specific when it comes to their substrates. I've observed how their active sites fit particular substrates like a glove, ensuring efficiency and precision in biochemical reactions. This precision is vital for maintaining cellular functions and studying metabolic pathways.
What Does It Mean to Say That an Enzyme Is Specific?
When I say an enzyme is specific, I mean it has a unique binding affinity for a particular substrate. This precision ensures that biochemical reactions occur efficiently and selectively, ultimately contributing to the precise regulation of metabolic processes.